Amy Sprague and Andy Freeberg
April 29, 2020
Part of the reason that most wind turbines look alike is because there is an established theory for how wind moves around the blades, which helped the industry converge on a design. No equivalent theory yet exists for cross-flow turbines because we still have a lot to learn about how fluids move around those blades. – Abigale Snortland
Unique challenges of marine energy
Mechanical engineering Ph.D. student Abigale Snortland is eager to get back to UW’s Harris Hydraulics Lab and its Alice C. Tyler flume, a long, fast-flowing channel of water that simulates river flows and tidal currents. Her team’s turbine research informs how to draw renewable energy from river, tidal and ocean currents.
These kinds of marine-generated energy have huge potential. Two other main sources of green energy, solar and wind, depend upon clear skies and consistent wind, which have significant downtimes. But marine energy benefits from steady and reliable water flows.
Despite this potential, marine energy projects have been hard to implement. Flowing water, with its forces, turbulence, sediments, salinity and algae, is tough on equipment. Maintenance may need to be done underwater during slack tides, the narrow window of time with weak tidal currents. Snortland’s work, for which she was recently awarded a prestigious National Science Foundation Graduate Research Fellowship, will apply aeronautics techniques to boost the performance of marine turbines and inform industry standards that reduce the overall challenges of such systems.
Abigale Snortland and Greg Talpey observe a cross-flow turbine in the Alice C. Tyler flume in the UW Harris Hydraulics Lab as part of a research collaboration among the Departments of Mechanical Engineering and Aeronautics & Astronautics and the Pacific Marine Energy Center.
Simpler turbines, not-so-simple physics
Snortland specifically researches how water moves through cross-flow marine turbines, which are different from the more familiar axial turbines, the structure of the common wind turbine. While axial turbines look like windmills, cross-flow turbines are structured more like a whisk, and, due to their relative mechanical simplicity, they provide some advantages in the harsh water environments.
She explains, “We are looking closely at cross-flow turbines because they have few moving parts and connections that could get corroded underwater. Unlike axial turbines in water, they don’t need to rotate to follow the direction of the changing tides and currents. They work regardless of which direction the tide is flowing. Even in wind, there are some applications where cross-flow turbines may be preferable to axial turbines.”
Cross-flow turbine
Axial-flow turbine
“Part of the reason that most wind turbines look alike is because there is an established theory for how wind moves around the blades, which helped the industry converge on a design. No equivalent theory yet exists for cross-flow turbines because we still have a lot to learn about how fluids move around those blades.”
While simpler for the corrosive waters and the variable directions of tides, the physics of these turbines are far less tidy, making it challenging to build a standard model. For a simple two-bladed spinning cross-flow turbine, one blade is moving with the flow while the other blade is going against it. This constant fight between the upstream and downstream blade results in strong moving vortices, a mess of turbulence and unsteady performance.
Brian Polagye leads the Pacific Marine Energy Center and co-advises Snortland. He says, “Generally, we have been able to make advances in performance through trial and error. But that can only get us so far. To advance the theories around turbine performance,” he continues, “we need to understand what is happening on each blade through Abigale’s experimental data.”
A UW research team tests a cross-flow turbine in Lake Washington with Governor Jay Inslee (at right). These tests complement the on-blade hydrodynamics research in which Snortland specializes.
Apply aerospace
To get beyond trial and error, Polagye teamed up with aeronautics and astronautics professor Owen Williams to co-advise Snortland. Williams is one of the UW’s leading experts in wind tunnel testing. He manages the UW aeronautics laboratory, one of two experimental wind tunnels on campus and has also built a small-scale supersonic wind tunnel in his lab in the bottom floor of Guggenheim Hall, which draws air from the large-scale Kirsten Wind Tunnel next door.
Williams has extensive experience with particle image velocimetry (PIV), a method of inserting small particles into a flow, lighting them with a laser and using a high-speed camera to photograph and chart the trajectories and speeds of those particles to map flows.
While it may seem counterintuitive to bring an aerospace expert into a marine energy project, water and air are both fluids, and the methods and principles of studying hydro- and aerodynamics are remarkably similar. Wind tunnels and water channels play by the same rules.
With Williams’ guidance, Snortland set up an experimental cross-flow turbine in the water flume and has been using PIV to analyze how the water is coming off the blades. She explains, “During a turbine rotation, the blades reach a certain angle to the incoming flow, and it causes little swirls that stay with the blade for a little bit and then drop off. This separation causes a dip in performance. Until now, we haven’t been able to see exactly what is happening on the blade. As we get more data, we can finally explain why small changes we make in the turbine setup affect performance.”
Data from Snortland and her team won’t just make turbines better; it’ll also make them smarter. Significant energy can be gained by improving the controls on the turbines, using knowledge of hydrodynamics to predict when to boost a turbine to keep it from stalling out or dampen it to keep from spinning too fast.
Snortland uses particle image velocimetry (PIV) to visualize the hydrodynamics of turbine blades. This research will advance our understanding of the conditions of efficient cross-flow turbine operation to inform new designs.
The goal: Remote energy systems worth the investment
The most immediate application of this research is to improve the prospects of self-contained remote energy systems. These systems could persistently and renewably power scientific equipment in the ocean or remote coastal villages. ME alumnus Ryan Tyler is an engineer at Ocean Renewable Power Company which has deployed a pilot cross-flow turbine system in the remote village of Igiugig, Alaska.
Tyler notes that recent improvements based on hydrodynamic data, like what Snortland is collecting, has allowed them to double the output of their systems. Not only will Snortland’s research further improve industry performance, but it will help inform and standardize the industry designs. “Ultimately,” he says, “this data will advance the whole field by bringing down design and production costs while raising performance, like what we’ve seen for the wind industry. With cheaper manufacturing and higher energy output, the economics of marine energy fall into place.”
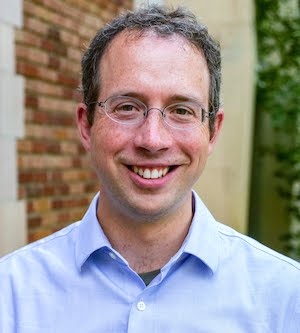
Brian Polagye
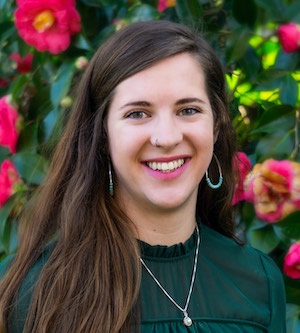
Abigale Snortland

Owen Williams
Video: Commissioning the Tidal Turbine Testing System on testing a full-scale cross-flow turbine prototype on the UW's research vessel Russell Davis Light in Lake Washington.
Learn more about the Pacific Marine Energy Center at pmec.us.